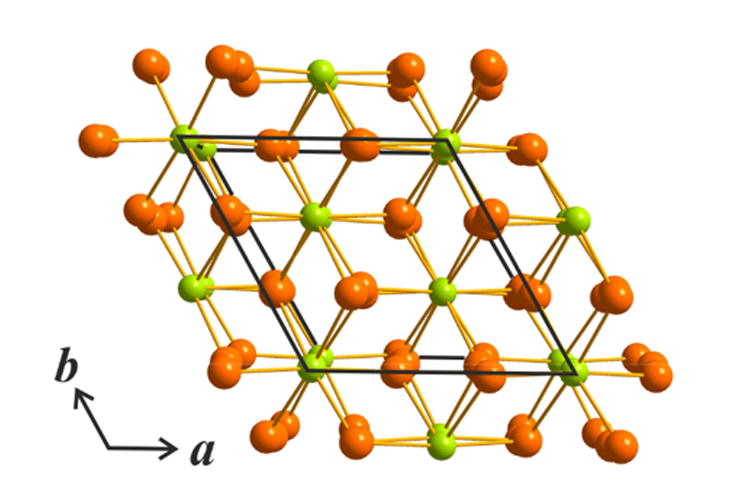
Reducing the dimensionality of condensed matter often leads to exceptional electronic, optical, and magnetic properties that enable new high-tech applications. Two-dimensional layered materials characterized by some interlayer bonds realized through very weak van der Waals (vdW) forces show an increasingly attractive appeal to scientists working in the fields of basic physics, material science and engineering. Slabs of atomic layers can be easily exfoliated by breaking the vdW bonds with little damage to both the extracted slab and the remaining structure. Novel physics and engineering of new ultrathin devices may be explored by exploiting the material properties of the slabs in isolation or by mixing and matching them to create new structures with atomically thin heterojunctions.
VI3 has received significant attention only in the past year [3-6]. It is known as a ferromagnet below TC 50K with complex magnetocrystalline anisotropy [4,6]. Limited unambiguous information on VI3 crystal structure, which has a decisive role for anisotropy, motivated us for a thorough structure study within the temperature interval 5 – 300 K. It was only known that VI3 has at room temperature a rhombohedral (trigonal) structure shown in Fig. 1 which transforms at Ts = 79 K to the low-temperature monoclinic crystal symmetry C2/c [3,4].
Fig. 1: Schematic picture of the room-temperature rhombohedral structure of VI3
Fig. 3: Evolution of (2 2 15) diffraction peaks of the VI3 single crystal in the temperature intervals from 78 to 80 K.
Fig. 2: Evolution of (2 2 15) diffraction peaks of the VI3 single crystal in the temperature intervals from 26 to 38 K.
Our detailed measurement and analysis of more than 2000 reflections from a VI3 single crystal at 250 K confirmed the existence of the room-temperature trigonal structure of the R-3 (148) space group. The common structural motif of TX3 compounds is a honeycomb net of transition-metal cations as shown in Fig. 1. The VI3 RT trigonal structure of the BiI3 type is characterized by the ABC layer stacking sequence. The subsequent layers are shifted along one of the V-V bonds. The honeycomb net is regular due to the three-fold symmetry. When decreasing temperature the (hhl) X-ray diffraction peaks suddenly split at temperatures between 79 and 78 K (see Fig. 2). This result unambiguously confirms that VI3 undergoes upon cooling a structure phase transition between the HT trigonal and the LT monoclinic phase at Ts = 79 K. In addition, the transition temperature Ts has been unexpectedly found by specific-heat measurements to decrease in magnetic fields applied along the trigonal c-axis.
Another surprise has been observed upon cooling VI3 across 32 K, in particular further splitting of some diffraction peaks indicating further lowering the crystal symmetry from monoclinic to triclinic. We associated this transition with the transformation of the ferromagnetic structure below 36 K, which has been reported by Gati et al. [5] from a thorough NMR study. The connections of structural transitions with magnetic phenomena in VI3 suggest a considerable role of magnetoelastic interactions in physics of this compound. This idea can be further specified when knowing microscopic aspects of the magnetic structures which are most probably not simple. Canted ferromagnetic structures have been suggested in previous papers [4,5]. Therefore, extensive investigations focused on details of the magnetism of VI3 are ongoing in MGML.
[1] W.-B. Zhang, Q. Qu, P. Zhu, and C.-H. Lam, J. Mater. Chem. C3, 12457 (2015).
[2] J. L. Lado and J. Fernández-Rossier, 2D Mater. 4, 035002 (2017).
[3] S. Son, M. J. Coak, N. Lee, J. Kim, T. Y. Kim, H. Hamidov, H. Cho, C. Liu, D. M. Jarvis, P. A. C. Brown, Jae Hoon Kim, Cheol-Hwan Park, Daniel I. Khomskii, Siddharth S. Saxena, and J-G. Park, Phys. Rev. B 99, 041402(R) (2019).
[4] T. Kong, K. Stolze, E. I. Timmons, J. Tao, D. Ni, S. Guo, Z. Yang, R. Prozorov, and R. J. Cava, Adv. Mater. 1808074 (2019).
[5] E. Gati, Y. Inagaki, T. Kong, R. J. Cava, Y. Furukawa, P.C. Canfield, and S. L. Bud’ko, Phys. Rev. B 100, 094408 (2019).
[6] J. Yan, X. Luo, F. C. Chen, J. J. Gao, Z. Z. Jiang, G. C. Zhao, Y. Sun, H. Y. Lv, S. J. Tian, Q. W. Yin, H. C. Lei, W. J. Lu, P. Tong, W. H. Song, X. B. Zhu, and Y. P. Sun, Phys. Rev. B 100, 094402 (2019).
[7] P. Doležal, M. Kratochvílová, V. Holý, P. Čermák, V. Sechovský, M. Dušek, M. Míšek, T. Chakraborty, Y. Noda, Suhan Son, and Je-Geun Park, Phys. Rev. Materials 3, 121401(R) (2019).